The Cardiac Cycle
The Cardiac Cycle
Overview
The cardiac cycle describes the sequence of mechanical and electrical events that repeat with every heartbeat. It encompasses the periods of contraction (systole) and relaxation (diastole) of the atria and ventricles, enabling the heart to pump blood throughout the body efficiently.
Here are the basics:
Anatomy of the Heart
To understand the cardiac cycle, it's essential to familiarize yourself with the heart's structure:
- Atria: The upper two chambers of the heart - the left atrium (LA) and the right atrium (RA).
- Ventricles: The lower two chambers of the heart - the left ventricle (LV) and the right ventricle (RV).
- Aorta: The main artery that carries oxygenated blood from the left ventricle to the rest of the body.
- Pulmonary Artery: The vessel that carries deoxygenated blood from the right ventricle to the lungs for oxygenation.
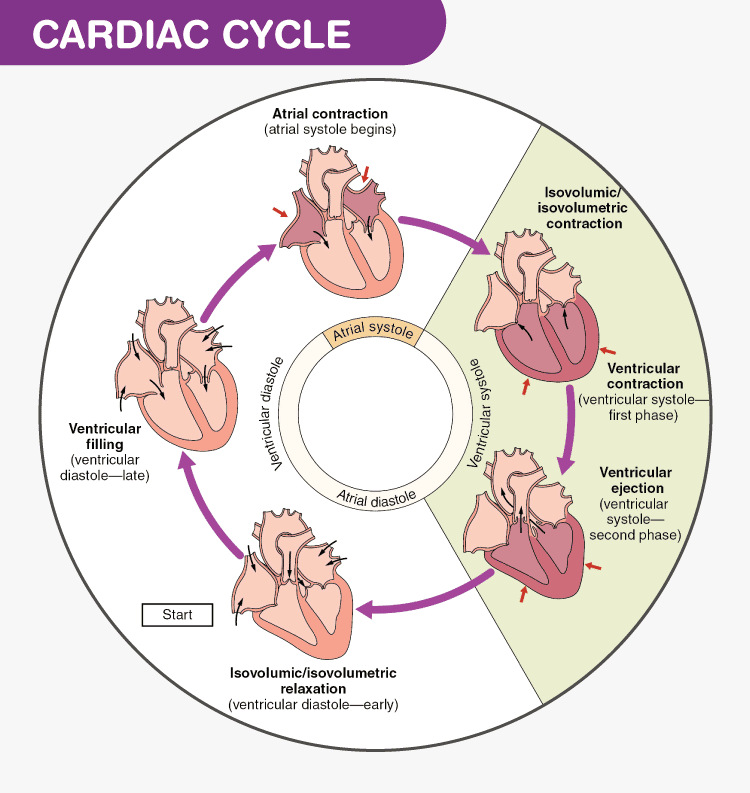
Phases of the Cardiac Cycle
The cardiac cycle consists of the following phases:
1. Atrial Systole
During atrial systole, the atria contract, pushing blood into the ventricles. This phase is crucial as it tops off the ventricles with blood, ensuring they are adequately filled before they contract.

2. Isovolumetric Contraction
This short phase occurs as the ventricles begin to contract, but all heart valves are closed. The pressure within the ventricles rises, but no blood is ejected yet.
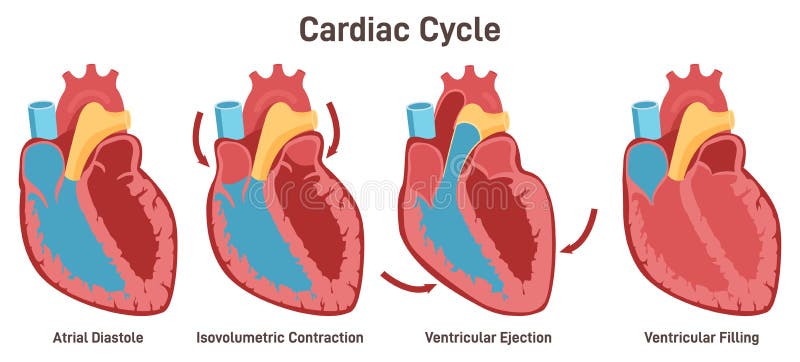
3. Ventricular Systole
During ventricular systole, the ventricles contract forcefully, causing the blood to be pumped out of the heart. The right ventricle sends blood through the pulmonary artery to the lungs, while the left ventricle sends blood through the aorta to the rest of the body.

4. Isovolumetric Relaxation
This phase occurs when the ventricles relax, but all valves are closed. Ventricular pressure falls, but no blood enters the ventricles at this time.
5. Ventricular Diastole
During ventricular diastole, the ventricles relax, and the heart valves open to allow blood to flow from the atria into the ventricles, preparing for the next cycle.
Understanding these phases is fundamental for comprehending heart function and diagnosing various cardiac disorders.
Let's do it again, this time in more depth:
Phase 0: Depolarization
- Ion Movement: Rapid influx of sodium ions (Na+).
- Mechanism: This phase is characterized by the rapid opening of fast voltage-gated sodium channels in response to a stimulus that depolarizes the membrane to a threshold level. Sodium ions rush into the cell, causing the membrane potential to rise quickly from around -90 mV to approximately +20 mV.
- Significance: This phase is responsible for the rapid depolarization that triggers the contraction of the cardiac muscle.
Phase 1: Initial Repolarization
- Ion Movement: Transient outward potassium current (K+).
- Mechanism: After the peak of Phase 0, some sodium channels inactivate, and voltage-gated potassium channels open briefly, allowing potassium to leave the cell. This creates a slight dip in the membrane potential, initiating repolarization.
- Significance: This phase is short and sets the stage for the plateau phase by partially repolarizing the cell.
Phase 2: Plateau Phase
- Ion Movement: Calcium influx (Ca++) and continued potassium efflux (K+).
- Mechanism: During this phase, L-type calcium channels open, allowing calcium to enter the cell. The calcium influx is balanced by the continued efflux of potassium, leading to a plateau in the membrane potential around 0 mV.
- Significance: The plateau phase prolongs the action potential, ensuring that the heart muscle has sufficient time to contract and pump blood effectively. This phase is critical for the contraction of the cardiac muscle (excitation-contraction coupling).
Phase 3: Rapid Repolarization
- Ion Movement: Increased potassium efflux (K+).
- Mechanism: The calcium channels close, and additional potassium channels open, increasing the efflux of potassium out of the cell. This leads to a rapid repolarization of the membrane potential back toward the resting potential.
- Significance: This phase restores the cell's membrane potential to its resting state, preparing the cell for the next action potential.
Phase 4: Resting Membrane Potential
- Ion Movement: Stable potassium current (K+).
- Mechanism: During this phase, the cell is at rest, with a stable membrane potential around -90 mV. The sodium-potassium ATPase pump and potassium leak channels maintain this resting potential by actively transporting sodium out of the cell and potassium into the cell.
- Significance: This phase represents the diastolic period when the heart muscle is relaxed and filling with blood. It ensures the cell is ready to respond to the next depolarizing stimulus.
Pacemaker Cells (SA and AV Nodes)
In pacemaker cells, such as those in the SA and AV nodes, the phases differ slightly:
- Phase 4: In pacemaker cells, Phase 4 is not stable. Instead, it shows a slow depolarization due to the "funny current" (If) where sodium and potassium ions gradually leak in and out, respectively, until the threshold is reached.
- Phase 0: Depolarization in pacemaker cells is primarily due to the influx of calcium ions (Ca++) through L-type calcium channels, resulting in a slower upstroke compared to ventricular myocytes.
- Phase 3: Repolarization is primarily due to potassium efflux (K+), similar to ventricular myocytes.
Pacemaker cells do not have a true Phase 1 or 2.
Summary of Phases:
- Phase 0: Rapid depolarization (Na+ influx in myocytes, Ca++ influx in pacemaker cells).
- Phase 1: Initial repolarization (transient K+ efflux).
- Phase 2: Plateau phase (Ca++ influx and K+ efflux).
- Phase 3: Rapid repolarization (increased K+ efflux).
- Phase 4: Resting membrane potential (stable K+ current in myocytes, slow depolarization in pacemaker cells).
Here's a fun AI song: